Originally posted on Microwave Journal
Written by Phil Lambert, Senior Customer Solutions Engineer at Fortify
In many microwave and millimeter-wave (mmW) applications, there is a drive to improve the performance of the communication or sensing system, to either stay competitive or exceed the range, throughput, accuracy, frequency range, agility, or other desirable aspect of the system. As microwave/mmW design and function dictates or otherwise heavily influences the performance of these systems, it is clear that improving antenna performance or introducing beamsteering enhancements can often provide the needed enhancement.
However, traditional metallic antenna typologies are limited by certain phenomena in terms of their size, shape, and performance. A way to overcome these limitations is to use metastructure techniques with arrangements of metallic conductive structures in three-dimensional (3D) space. Given the nature of metallic conductors as a material, there are also limitations to the size, shape, performance, and manufacturability of metallic metastructure antennas. Conveniently, microwave/mmW metastructures can also be fabricated using dielectric materials, which in some cases, may be easier to machine or otherwise fabricate into complex 3D structures at the scale and resolution tolerances necessary to yield microwave/mmW antennas or lenses. Recent advancements in 3D printing dielectric materials has also opened doors to the types, cost, degrees of freedom, and time-scales associated with fabricating dielectric metastructures for small-/large-scale manufacturing.
A notable result of these advancements and new capabilities/materials is the ability to cost-effectively fabricate even extremely complex gradient-refractive index (GRIN) style dielectric antennas and lenses. GRIN dielectric lens (or lens antenna) are a type of dielectric metastructure with a continuous spatially graded index of refraction, which allows for some control of the electromagnetic radiation passing through the structure. In short, GRIN dielectric lenses/antennas can be used to dramatically alter the performance of an antenna by modifying/augmenting the gain, directivity, antenna pattern, steering angle, bandwidth, and other key antenna parameters.
Hence, GRIN dielectric lenses/antennas have been a hot topic of research for virtually every microwave/mmW application, from military/defense, aerospace/Space, to commercial telecommunications. There have even been several companies, products, and government/DoD grants using GRIN dielectric lens/antenna technology. This blog provides some discussion around recent (since 2018) research on GRIN dielectric lenses/antennas, and an overview of GRIN dielectric lenses/antennas being deployed or intended for practical use.
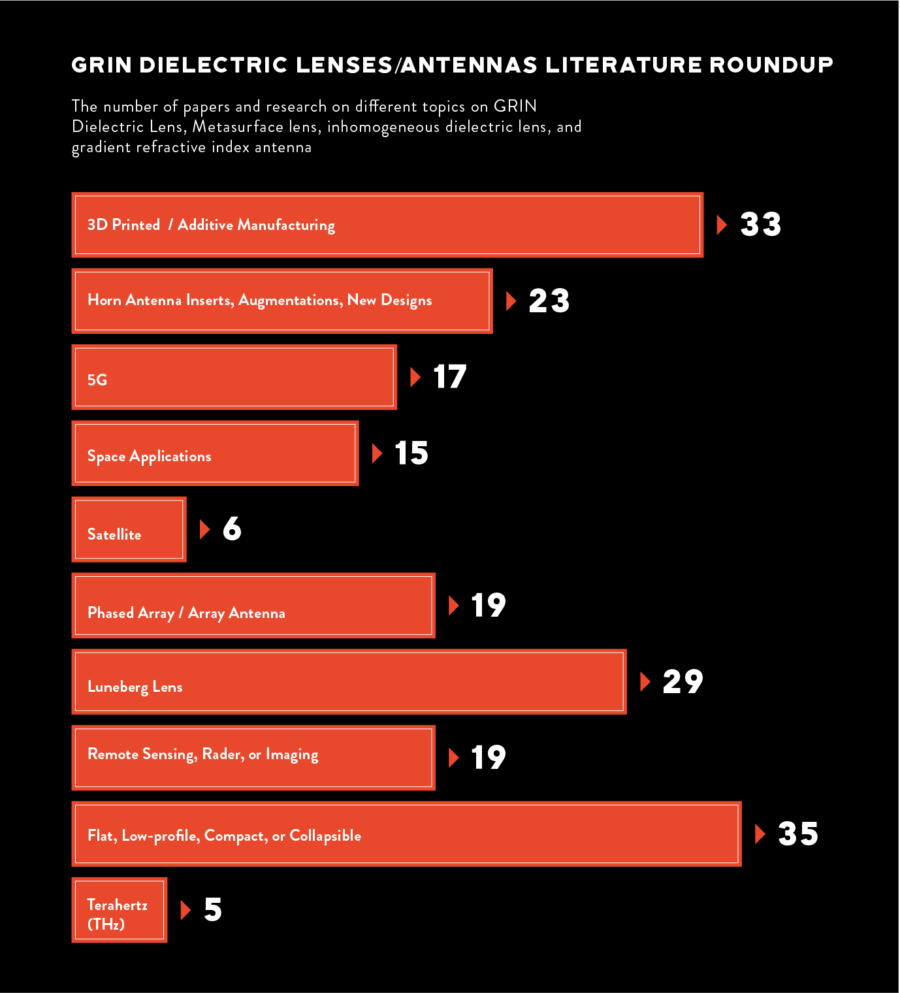
Research Literature Review Discussion
In general, a research review revealed that there are a few main authors/research groups publishing research on GRIN dielectric lense/antenna technology in recent years. Much of the research is focused on developing new types of GRIN lenses/antennas, augmenting horn antennas using GRIN dielectric lenses, augmenting phased array/array antennas, modeling and design methods of realizing GRIN dielectric lenses (including unit cell designs), and new GRIN dielectric lens designs that are intended to solve application specific challenges for 5G telecommunications, satellite communications, or various sensor applications.
Literature Review Categories
The following bullets list different categories of research and papers on GRIN Dielectric Lens, Metasurface lens, inhomogeneous dielectric lens, gradient refractive index antenna [1]–[103] which include:
- 3D Printed/Additive Manufacture [1]–[33]
- Illustrating this category is the research from [20] which discusses work from MIT Lincoln Laboratory on flat lens designs for antennas, matching networks, and filters using graded index composite materials.
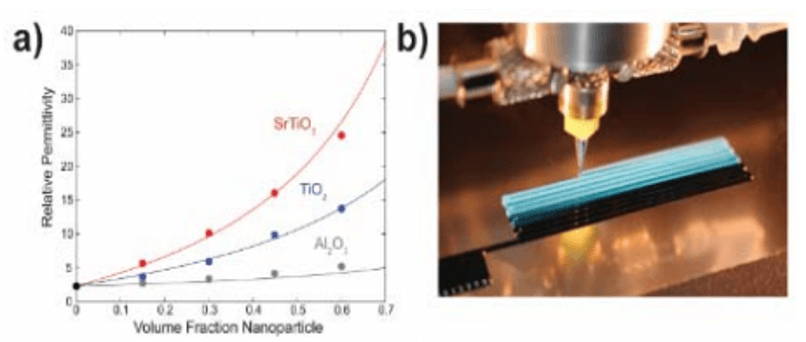
MIT-LL dielectric deposition technology. a) Range of relative permittivity that can be generated. Measured data are the dot-dot curves while the solid lines represent the model used to fit the measured data b) Active mixing nozzle printing a graded filament. Source [20]
- Horn Antenna inserts, augmentations, new designs [1], [8], [11], [12], [20], [23], [24], [30], [34]–[48]
- Demonstrating this category is the research from [35] which describes the use of conformal transformation optics to enhance the directivity of an H-plane horn antenna using GRIN materials.
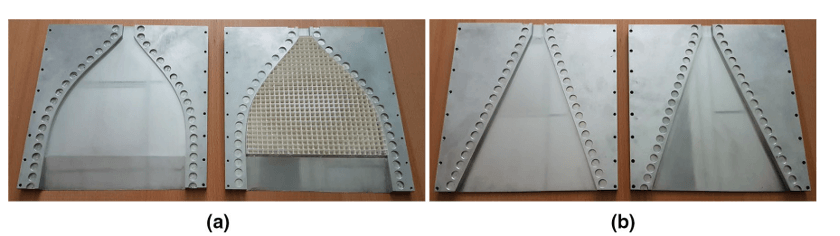
Photograph of (a) the H-plane horn lens prototype, and (b) the reference H-plane horn prototype.” Source [35]
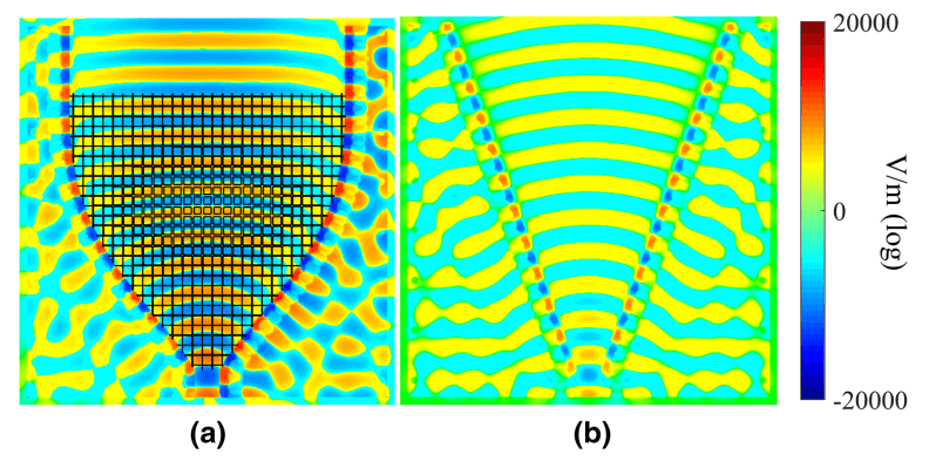
Simulated amplitude distribution of Ez for the two H-plane antennas: (a) horn lens, and (b) reference horn. The dimensions are LL = LH = 207 mm, WL = WH = 167 mm, HL = HH = 20 mm, and FL = 40 mm.”Source [35]
- 5G [7], [11], [18], [30], [32], [46], [49]–[59]
- Space Applications [23], [24], [26], [27], [31], [35], [44], [45], [48], [60]–[65]
- Satellite [26], [50], [66]–[69]
- Phased Array/Array Antenna [8], [10], [11], [14], [19], [23], [24], [26], [30], [34], [42], [49], [52], [63], [67], [69]–[72]
- Luneburg Lens [3], [5], [6], [8], [9], [12], [22], [26]–[28], [31], [32], [46], [53]–[55], [57]–[59], [61], [66], [67], [70], [73]–[78]
- An example of this type of research is [55] which discusses a 3D-printed wideband parallel-plate circularly polarized Luneburg lens antenna.
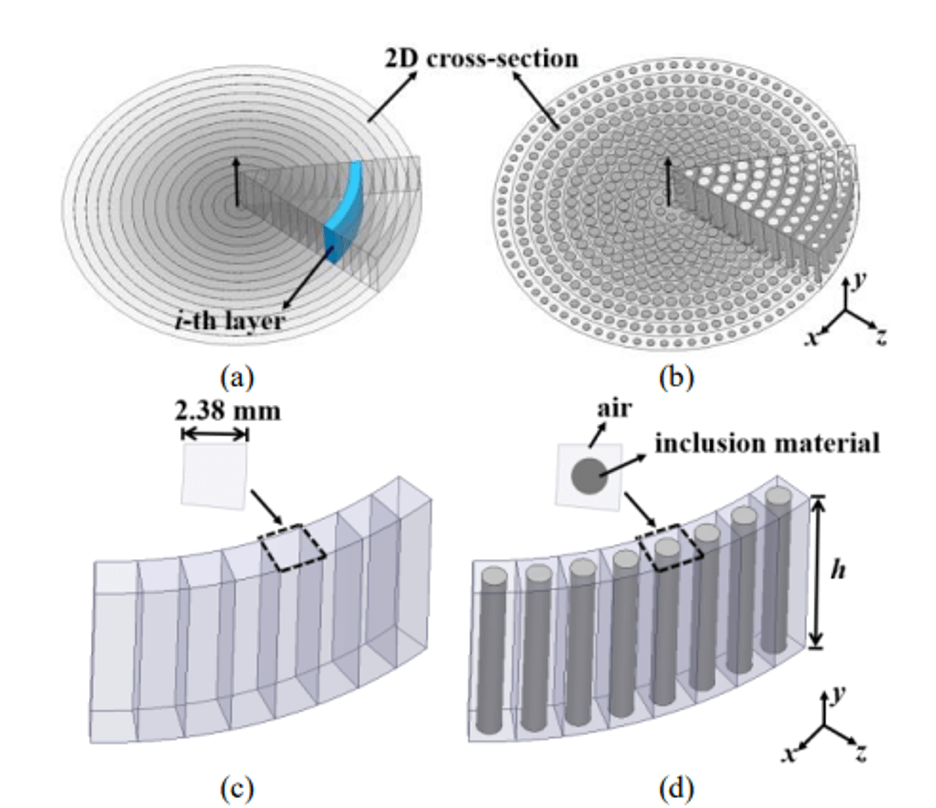
(a) Luneburg lens constructed by sweeping the 2D cross-section along the y-direction where darker color representing increasing permittivity. (b) LL’s structure constructed by dielectric posts. (c) & (d) i-th layer before and after inserting inclusion material into the unit cells (insets: unit cell). Source [55]
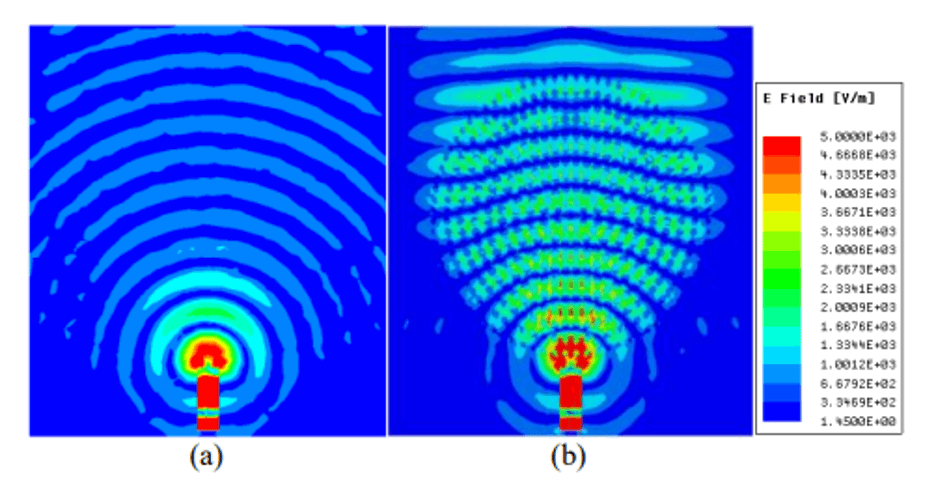
E-field distribution for Ex components at 28 GHz for a LL illuminated by an open-ended waveguide (a) before (b) after applying EMT. Source [55]
- Remote Sensing, Radar, or Imaging [3], [4], [16], [23], [24], [26], [53], [54], [79]–[82]
- Flat, Low-profile, Compact, or Collapsible [4], [10], [15], [20]–[24], [26], [27], [32], [33], [37], [39], [41], [49]–[51], [53], [54], [60], [67], [69], [72]–[76], [78], [81], [83]–[87]
- Terahertz (THz) [3], [21], [61], [73], [74]
Earlier research into GRIN lenses were often of the Luneberg type or inserts/augments for waveguide horn antennas. The results of much of this research were proof-of-concept type 3D objects, or demonstrations of gradient refractive index synthesis or manufacturing techniques. More recent research appears to be focusing on providing more practical dielectric lens designs in more compact form factors, some even collapsible, such as for satellite communications. Another large set of recent research focuses on improving the algorithms and design tools to yield GRIN and dielectric metasurface lenses that are more computationally efficient, accurate, or enable more straightforward unit cell design. Additionally, there has been a significant amount of research into integrating other types of structures alongside or including GRIN dielectric regions, such as absorbers/anti-reflective layers, reflectors, and complex conductive antenna structures. Other areas of interest in the body of GRIN dielectric research include the use of various nontraditional materials to realize GRIN structures, including fused-filament deposition (FDM) printable polymers, cold sintered ceramics/composites, and various other 3D printable materials. There is even a small but seemingly growing body of research focused on using GRIN dielectric structures for Terahertz (THz) communication experiments.
GRIN Dielectric Lenses In Action [104]–[109]
Advancement of dielectric lens/antenna for enhancement of array antenna and antenna structures housed in confined spaces has been of particular interest to the US Department of Defense (DoD). In particular, dielectric lenses/antennas capable of ultra-wideband (UWB) and high power operation while maintaining a compact profile (often flat) have appeared in small business innovation research (SBIR) grant abstracts [104,105,107-109]. The DoD applications appear to be predominately focused on enhanced radar and sensing technology. Given the relatively recent advancements of GRIN dielectric lens/antenna, there may be many additional applications in the process of development or even deployment where information regarding these GRIN applications isn’t publicly available.
There have been a few commercial products created using GRIN and Luneburg lens/antenna technology. The solutions that have publicly available information include passive radar, automotive radar, satellite communication arrays (space), satellite communication ground terminals, and multi-beam antennas for 5G telecommunication applications. The majority of these applications cover microwave/mmW frequencies, which is likely due to the frequency dependent size of dielectric lens and antenna structures, which become practical at microwave frequencies.\
GRIN dielectric lens/antenna technology is being heavily pursued in order to overcome the constraints of traditional antenna design and enhance microwave/mmW antenna performance beyond what is achievable with purely metallic conductive antennas. Upper microwave and mmW communication and sensing applications tend to suffer from higher
RF and atmospheric losses, and GRIN dielectric lens/antenna technology may provide a way to offset some of these losses by providing enhanced gain/directivity while minimizing the relatively Size, Weight, Power, and Cost (SWAP-C) associated with traditional higher gain antenna topologies. Another major area of possibility is the use of GRIN lenses/antennas to provide enhanced beamsteering capability for electronically steered array antennas. It appears likely to the author that within the next few years that there will be many more examples of GRIN dielectric lens/antenna technology deployed in a variety of applications, including military/aerospace, drone/Unmanned Aerial Vehicle (UAV) communication, satellite communications, and automotive radar.
Resources
[1] S. Zhang, Y. Vardaxoglou, W. Whittow, and R. Mittra, “3D-printed graded index lens for RF applications,” in 2016
International Symposium on Antennas and Propagation (ISAP), Oct. 2016, pp. 90–91.
[2] M. S. Anwar, H. Abufanas, and A. Bangert, “3D printed dielectric lens for the gain enhancement of a broadband antenna,”
Int. J. RF Microw. Comput.-Aided Eng., vol. 30, no. 4, Apr. 2020, doi: 10.1002/mmce.22115.
[3] J. Chen, M. Chen, and D. Fang, “Broad Terahertz Radial Perfectly Symmetric Gradient Honeycomb All-Dielectric Planar
Luneburg Lens,” in 2019 Thirteenth International Congress on Artificial Materials for Novel Wave Phenomena
(Metamaterials), Sep. 2019, p. X-083-X–085. doi: 10.1109/MetaMaterials.2019.8900839.
[4] J. Chen et al., “Ultrabroadband compact lens antenna with high performance based on a transmission gradient index
medium,” J. Phys. Appl. Phys., vol. 54, no. 17, p. 175101, Feb. 2021, doi: 10.1088/1361-6463/abddf9.
[5] J. Chen et al., “An all-dielectric 3D Luneburg lens constructed by common-vertex coaxial circular cones,” J. Phys. Appl.
Phys., vol. 53, no. 1, p. 015110, Oct. 2019, doi: 10.1088/1361-6463/ab3fb8.
[6] J. Chen et al., “Ultrabroadband Three-Dimensional Printed Radial Perfectly Symmetric Gradient Honeycomb All-Dielectric
Dual-Directional Lightweight Planar Luneburg Lens,” ACS Appl. Mater. Interfaces, vol. 10, no. 44, pp. 38404–38409, Nov.
2018, doi: 10.1021/acsami.8b11239.
[7] N. Garcia and J. Chisum, “Reduced dimensionality optimizer for efficient design of wideband millimeter-wave 3D
metamaterial GRIN lenses,” Microw. Opt. Technol. Lett., vol. 63, no. 5, pp. 1372–1376, 2021, doi: 10.1002/mop.32755.
[8] I. Goode and C. E. Saavedra, “3D Printed 18 GHz to 28 GHz Horn Antenna and Gradient Index of Refraction Lens,” in 2021
XXXIVth General Assembly and Scientific Symposium of the International Union of Radio Science (URSI GASS), Aug 2021, pp.
1–4. doi: 10.23919/URSIGASS51995.2021.9560278.
[9] I. Grigoriev, I. Munina, and D. Zelenchuk, “3D printed Ku band cylindrical Luneburg lens,” J. Phys. Conf. Ser., vol. 2015, no. 1,
- 012095, Nov. 2021, doi: 10.1088/1742-6596/2015/1/012095.
[10] A. M. Hegazy, M. A. Basha, and S. Safavi-Naeini, “3D-Printed Scanning Dielectric Lens Antenna,” in 2019 IEEE International
Symposium on Antennas and Propagation and USNC-URSI Radio Science Meeting, Jul. 2019, pp. 1991–1992. doi:
10.1109/APUSNCURSINRSM.2019.8889020.
[11] C. A. M. Hernandez, M. Elmansouri, and D. S. Filipovic, “High-Directivity Beam-Steerable Lens Antenna for Simultaneous
Transmit and Receive,” in 2019 IEEE International Symposium on Phased Array System Technology (PAST), Oct. 2019, pp.
1–5. doi: 10.1109/PAST43306.2019.9020904.
[12] K. V. Hoel, S. Kristoffersen, M. Ignatenko, and D. Filipovic, “Half ellipsoid luneburg GRIN dielectric lens loaded double
ridged horn antenna,” in 12th European Conference on Antennas and Propagation (EuCAP 2018), Apr. 2018, pp. 1–5. doi:
10.1049/cp.2018.0523.
[13] D. Isakov, Y. Wu, B. Allen, C. J. Stevens, and P. S. Grant, “3D-printed optical devices with refractive index control for
microwave applications,” TechConnect Briefs, vol. 4, no. Informatics, Electronics and Microsystems: TechConnect Briefs
2018, 2018, Accessed: Jan. 06, 2022. [Online]. Available: https://ora.ox.ac.uk/objects/uuid:2f2a0b20-6b00-424b-8b9b-
7e56340aa6c8
[14] A. Massaccesi, P. Pirinoli, and J. C. Vardaxoglou, “A Multilayer Unit-cell for Perforated Dielectric Transmitarray Antennas,”
in 2018 IEEE International Symposium on Antennas and Propagation USNC/URSI National Radio Science Meeting, Jul. 2018,
- 263–264. doi: 10.1109/APUSNCURSINRSM.2018.8608712.
[15] J. M. Monkevich and G. P. Le Sage, “Design and Fabrication of a Custom-Dielectric Fresnel Multi-Zone Plate Lens Antenna
Using Additive Manufacturing Techniques,” IEEE Access, vol. 7, pp. 61452–61460, 2019, doi:
10.1109/ACCESS.2019.2916077.
[16] A. D. O’Neill, J. C. Davidson, T. J. Kane, and R. M. Narayanan, “Additive manufacturing for microwave and millimeter-wave
antennas: a summary of current technology and experimentation,” in Image Sensing Technologies: Materials, Devices,
Systems, and Applications VI, May 2019, vol. 10980, pp. 161–177. doi: 10.1117/12.2519549.
[17] Y. Oh, V. T. Bharambe, J. J. Adams, D. Negro, and E. MacDonald, “Design of a 3D Printed Gradient Index Lens Using High
Permittivity Ceramic,” 2019, Accessed: Jan. 04, 2022. [Online]. Available:
https://ieeexplore.ieee.org/servlet/opac?punumber=9329358
[18] J.-M. Poyanco, F. Pizarro, and E. Rajo-Iglesias, “3D-printed dielectric GRIN planar wideband lens antenna for 5G
applications,” in 2021 15th European Conference on Antennas and Propagation (EuCAP), Mar. 2021, pp. 1–4. doi:
10.23919/EuCAP51087.2021.9411342.
[19] R. Rojas and P. Bluem, “Graded Index Dielectric Superstrate for Phased Array Scan Compensation,” in 2020 IEEE
International Symposium on Antennas and Propagation and North American Radio Science Meeting, Jul. 2020, pp. 1291–
- doi: 10.1109/IEEECONF35879.2020.9329716.
[20] R. Rojas, P. Bluem, I. Osaretin, and B. Duncan, “Antennas and RF Components Designed with Graded Index Composite
Materials,” in 2020 International Applied Computational Electromagnetics Society Symposium (ACES), Jul. 2020, pp. 1–2.
doi: 10.23919/ACES49320.2020.9196038.
[21] M. Sedaghat, V. Nayyeri, M. Soleimani, and O. M. Ramahi, “New Approaches to Design and Realization of Dielectric GRIN
Flat Lenses,” in 2018 Fifth International Conference on Millimeter-Wave and Terahertz Technologies (MMWaTT), Dec.
2018, pp. 49–53. doi: 10.1109/MMWaTT.2018.8661237.
[22] W. Shao and Q. Chen, “A Novel Planar Perforated Hyperbolic Secant Lens Antenna for Milli-Wave Applications,” in 2021
International Symposium on Antennas and Propagation (ISAP), Oct. 2021, pp. 1–2. doi:
10.23919/ISAP47258.2021.9614523.
[23] S. G. Wirth, I. L. Morrow, and I. Horsfall, “Near-Field Microwave Imaging using a Polarimetric Array of 3D Printed
Antennas and Lenses,” p. 65 (6 pp.)-65 (6 pp.), Jan. 2018, doi: 10.1049/cp.2018.1486.
[24] S. G. Wirth and I. L. Morrow, “Near-Field Microwave Sensor Composed of 3D Printed Antennas and Lenses,” in 2018 IEEE
MTT-S International Conference on Numerical Electromagnetic and Multiphysics Modeling and Optimization (NEMO), Aug.
2018, pp. 1–4. doi: 10.1109/NEMO.2018.8503209.
[25] J. Xu et al., “Multi-objective Optimization of Directivity-Enhancing GRIN Lenses,” in 2020 IEEE International Symposium on
Antennas and Propagation and North American Radio Science Meeting, Jul. 2020, pp. 1977–1978. doi:
10.1109/IEEECONF35879.2020.9329890.
[26] S. Biswas, “Design and additive manufacturing of broadband beamforming lensed antennas and load bearing conformal
antennas,” Thesis, University of Delaware, 2019. Accessed: Jan. 04, 2022. [Online]. Available:
https://udspace.udel.edu/handle/19716/24999
[27] S. Biswas et al., “Realization of modified Luneburg lens antenna using quasi-conformal transformation optics and additive
manufacturing,” Microw. Opt. Technol. Lett., vol. 61, no. 4, pp. 1022–1029, 2019, doi: 10.1002/mop.31696.
[28] S. Biswas and M. Mirotznik, Customized shaped Luneburg Lens Antenna Design by Additive Fabrication. 2018. doi:
10.1109/ANTEM.2018.8572910.
[29] H. Giddens and Y. Hao, “Multibeam Graded Dielectric Lens Antenna From Multimaterial 3-D Printing,” IEEE Trans.
Antennas Propag., vol. 68, no. 9, pp. 6832–6837, Sep. 2020, doi: 10.1109/TAP.2020.2978949.
[30] B. Horn, S. Yee, H. ElBidweihy, and D. Mechtel, “Additively Manufactured Mobile Device Lens Case for 5G Antenna Gain,”
in 2021 IEEE 71st Electronic Components and Technology Conference (ECTC), Jun. Mechtel, “Additively Manufactured
Mobile Device Lens Case for 5G Antenna Gain,” in 2021 IEEE 71st Electronic Components and Technology Conference
(ECTC), Jun. 2021, pp. 1721–1731. doi: 10.1109/ECTC32696.2021.00272.doi:
10.1109/ECTC32696.2021.00272.
[31] Z. Larimore, S. Jensen, A. Good, A. Lu, J. Suarez, and M. Mirotznik, “Additive Manufacturing of Luneburg Lens Antennas
Using Space-Filling Curves and Fused Filament Fabrication,” IEEE Trans. Antennas Propag., vol. 66, no. 6, pp. 2818–2827,
Jun.
Jun. 2018, doi: 10.1109/TAP.2018.2823819.
[32] W. Shao, H. Sato, X. Li, K. K. Mutai, and Q. Chen, “Perforated extensible 3-D hyperbolic secant lens antenna for directive
antenna applications using additive manufacturing,” Opt. Chen, “Perforated extensible 3-D hyperbolic secant lens
antenna for directive
antenna applications using additive manufacturing,” Opt. Express, vol. 29, no. 12, pp. 18932–18949, Jun. 2021, doi:
10.1364/OE.426824.2021, doi:
10.1364/OE.426824.
[33] S. Zhang, R. K. Arya, W. G. Whittow, D. Cadman, R. Mittra, and J. C. Vardaxoglou, “Ultra-Wideband Flat Metamaterial GRIN
Lenses Assisted With Additive Manufacturing Technique,” IEEE Trans. Vardaxoglou, “Ultra-Wideband Flat Metamaterial
GRIN
Lenses Assisted With Additive Manufacturing Technique,” IEEE Trans. Antennas Propag., vol. 69, no. 7, pp. 3788–3799, Jul.
2021, doi: 10.1109/TAP.2020.3044586.
[34] A. K. Baghel, S. S. Kulkarni, and S. K. Nayak, “Far-Field Wireless Power Transfer Using GRIN Lens Metamaterial at GHz
Frequency,” IEEE Microw. Nayak, “Far-Field Wireless Power Transfer Using GRIN Lens Metamaterial at GHz
Frequency,” IEEE Microw. Wirel. Compon. Lett., vol. 29, no. 6, pp. 424–426, Jun. 2019, doi: 10.1109/LMWC.2019.2912056.
[35] H. Eskandari, J. L. Albadalejo-Lijarcio, O. Zetterstrom, T. Tyc, and O. Quevedo-Teruel, “H-plane horn antenna with
enhanced directivity using conformal transformation optics,” Sci. Quevedo-Teruel, “H-plane horn antenna with
enhanced directivity using conformal transformation optics,” Sci. Rep., vol. 11, no. 1, p. 14322, Jul. 2021, doi:
10.1038/s41598-021-93812-6.2021, doi:
10.1038/s41598-021-93812-6.
[36] H. Eskandari, T. Tyc, J. L. Albadalejo-Lijarcio, O. Zetterstrom, and O. Quevedo-Teruel, “Design of an Impedance-Matched
Horn Antenna with Enhanced Directivity Using Conformal Transformation Optics,” in 2020 14th European Conference on
Antennas and Propagation (EuCAP), Mar. Quevedo-Teruel, “Design of an Impedance-Matched
Horn Antenna with Enhanced Directivity Using Conformal Transformation Optics,” in 2020 14th European Conference on
Antennas and Propagation (EuCAP), Mar. 2020, pp. 1–4. doi: 10.23919/EuCAP48036.2020.9135870.
[37] Y. He and G. V. Eleftheriades, “Matched, Low-Loss, and Wideband Graded-Index Flat Lenses for Millimeter-Wave
Applications,” IEEE Trans. Eleftheriades, “Matched, Low-Loss, and Wideband Graded-Index Flat Lenses for Millimeter-
Wave
Applications,” IEEE Trans. Antennas Propag., vol. 66, no. 3, pp. 1114–1123, Mar. 2018, doi: 10.1109/TAP.2018.2790173.
[38] K. V. Hoel, M. Ignatenko, S. Kristoffersen, E. Lier, and D. S. Filipovic, “3-D Printed Monolithic GRIN Dielectric-Loaded
Double-Ridged Horn Antennas,” IEEE Trans. Filipovic, “3-D Printed Monolithic GRIN Dielectric-Loaded
Double-Ridged Horn Antennas,” IEEE Trans. Antennas Propag., vol. 68, no. 1, pp. 533–539, Jan. 2020, doi:
10.1109/TAP.2019.2938563.2020, doi:
10.1109/TAP.2019.2938563.
[39] Q.-W. Lin and H. Wong, “A Low-Profile and Wideband Lens Antenna Based on High-Refractive-Index Metasurface,” IEEE
Trans. Antennas Propag., vol. 66, no. 11, pp. 5764–5772, Nov. 2018, doi: 10.1109/TAP.2018.2866528.
[40] K. Liu, Y. Ge, and C. Lin, “A Compact Wideband High-Gain Metasurface-Lens-Corrected Conical Horn Antenna,” IEEE
Antennas Wirel. Propag. Lett., vol. 18, no. 3, pp. 457–461, Mar. 2019, doi: 10.1109/LAWP.2019.2894037.
[41] S. L. Liu, X. Q. Lin, J. Y. Lan, and X. B. He, “W-band flat lens antenna with compact size and broadband performance,” IET
Microw. Antennas Propag., vol. 14, no. 10, pp. 1047–1052, 2020, doi: 10.1049/iet-map.2019.0654.
[42] B. P. Mishra, S. Sahu, S. K. S. Parashar, and S. K. Pathak, “A compact wideband and high gain GRIN metamaterial lens
antenna system suitable for C, X, Ku band application,” Optik, vol. 165, pp. 266–274, Jul. 2018, doi:
10.1016/j.ijleo.2018.03.092.
[43] J. Pourahmadazar, R. Karimian, M. D. Ardakani, Sh. Ahmadi, and M. Zaghloul, “Alumide as a Nonmagnetic Fresnel Zone
Lens at 30 GHz,” in 2021 XXXIVth General Assembly and Scientific Symposium of the International Union of Radio Science
(URSI GASS), Aug. 2021, pp. 1–3. doi: 10.23919/URSIGASS51995.2021.9560347.
[44] H. W. Tian, W. Jiang, X. Li, Z. P. Chen, and T. J. Cui, “An Ultrawideband and High-Gain Antenna Based on 3-D Impedance-
Matching Metamaterial Lens,” IEEE Trans. Antennas Propag., vol. 69, no. 6, pp. 3084–3093, Jun. 2021, doi:
10.1109/TAP.2020.3037751.
[45] L.-J. Wang, Q.-H. Chen, F.-L. Yu, and X. Gao, “High-performance lens antenna using high refractive index metamaterials,”
Chin. Phys. B, vol. 27, no. 8, p. 087802, Aug. 2018, doi: 10.1088/1674-1056/27/8/087802.
[46] X. Wang, Y. Cheng, and Y. Dong, “A Wideband PCB-Stacked Air-Filled Luneburg Lens Antenna for 5G Millimeter-Wave
Applications,” IEEE Antennas Wirel. Propag. Lett., vol. 20, no. 3, pp. 327–331, Mar. 2021, doi:
10.1109/LAWP.2021.3049432.
[47] W. Yu, L. Peng, Y. Liu, Q. Zhao, X. Jiang, and S. Li, “An Ultrawideband and High-Aperture-Efficiency All-Dielectric Lens
Antenna,” IEEE Antennas Wirel. Propag. Lett., vol. 20, no. 12, pp. 2442–2446, Dec. 2021, doi:
10.1109/LAWP.2021.3114159.
[48] N. Zhang, W. X. Jiang, H. F. Ma, W. X. Tang, and T. J. Cui, “Compact High-Performance Lens Antenna Based on Impedance-
Matching Gradient-Index Metamaterials,” IEEE Trans. Antennas Propag., vol. 67, no. 2, pp. 1323–1328, Feb. 2019, doi:
10.1109/TAP.2018.2880115.
[49] S. Cho and H.-J. Song, “Ultra-low Power Beamforming Wideband OFDM Signal with Active GRIN Lens at 19 GHz,” in 2020
IEEE International Symposium on Antennas and Propagation and North American Radio Science Meeting, Jul. 2020, pp.
583–584. doi: 10.1109/IEEECONF35879.2020.9329701.
[50] N. C. Garcia and J. D. Chisum, “High-Efficiency, Wideband GRIN Lenses With Intrinsically Matched Unit Cells,” IEEE Trans.
Antennas Propag., vol. 68, no. 8, pp. 5965–5977, Aug. 2020, doi: 10.1109/TAP.2020.2990289.
[51] F. Maggiorelli, A. Paraskevopoulos, J. C. Vardaxoglou, M. Albani, and S. Maci, “Profile Inversion and Closed Form
Formulation of Compact GRIN Lenses,” IEEE Open J. Antennas Propag., vol. 2, pp. 315–325, 2021, doi:
10.1109/OJAP.2021.3059468.
[52] Z. Qu, S.-W. Qu, Z. Zhang, S. Yang, and C. H. Chan, “Wide-Angle Scanning Lens Fed by Small-Scale Antenna Array for 5G in
Millimeter-Wave Band,” IEEE Trans. Antennas Propag., vol. 68, no. 5, pp. 3635–3643, May 2020, doi:
10.1109/TAP.2020.2967086.
[53] Y. Su and Z. N. Chen, “A Flat Dual-Polarized Transformation-Optics Beamscanning Luneburg Lens Antenna Using PCB-
Stacked Gradient Index Metamaterials,” IEEE Trans. Antennas Propag., vol. 66, no. 10, pp. 5088–5097, Oct. 2018, doi:
10.1109/TAP.2018.2858209.
[54] Y. Su and Z. N. Chen, “A Radial Transformation-Optics Mapping for Flat Ultra-Wide-Angle Dual-Polarized Stacked GRIN
MTM Luneburg Lens Antenna.” https://ieeexplore.ieee.org/abstract/document/8649598/ (accessed Jan. 05, 2022).
[55] C. Wang, J. Wu, and Y.-X. Guo, “A 3-D-Printed Wideband Circularly Polarized Parallel-Plate Luneburg Lens Antenna,” IEEE
Trans. Antennas Propag., vol. 68, no. 6, pp. 4944–4949, Jun. 2020, doi: 10.1109/TAP.2019.2955222.
[56] D. Wang et al., “Temperature Stable Cold Sintered (Bi0.95Li0.05)(V0.9Mo0.1)O4-Na2Mo2O7 Microwave Dielectric
Composites,” Materials, vol. 12, no. 9, Art. no. 9, Jan. 2019, doi: 10.3390/ma12091370.
[57] X. Wang and Y. Dong, “A PCB-stacked air-filled Luneburg reflector lens antenna for 5G millimeter wave applications,”
Microw. Opt. Technol. Lett., vol. 63, no. 12, pp. 3041–3046, 2021, doi: 10.1002/mop.33016.
[58] X. Wang and Y. Dong, “Wideband Multi-Beam Luneburg Lens Antenna Based on Effective Medium Theory,” in 2021
International Conference on Microwave and Millimeter Wave Technology (ICMMT), May 2021, pp. 1–3. doi:
10.1109/ICMMT52847.2021.9617942.
[59] X. Wang, Y. Pan, and Y. Dong, “An E-Plane-Focused Triple-Layer Multi-beam Luneburg Lens Antenna for 5G Millimeter-
Wave Applications,” IEEE Antennas Wirel. Propag. Lett., pp. 1–1, 2021, doi: 10.1109/LAWP.2021.3124129.
[60] F. Albarracin-Vargas, F. Vega, C. Kasmi, F. AlYafei, and C. Baer, “Dual Graded Index Dielectric Lens System for
Hyperthermia,” in 2020 XXXIIIrd General Assembly and Scientific Symposium of the International Union of Radio Science,
Aug. 2020, pp. 1–4. doi: 10.23919/URSIGASS49373.2020.9232223.
[61] Y. Amarasinghe et al., “Broadband wide-angle terahertz antenna based on the application of transformation optics to a
Luneburg lens,” Sci. Rep., vol. 11, no. 1, p. 5230, Mar. 2021, doi: 10.1038/s41598-021-84849-8.
[62] R. Cicchetti, V. Cicchetti, A. Faraone, and O. Testa, “A Class of Lightweight Spherical-Axicon Dielectric Lenses for High Gain
Wideband Antennas,” IEEE Access, vol. 9, pp. 151873–151887, 2021, doi: 10.1109/ACCESS.2021.3122575.
[63] J. Lei, J. Yang, X. Chen, Z. Zhang, G. Fu, and Y. Hao, “Experimental demonstration of conformal phased array antenna via
transformation optics,” Sci. Rep., vol. 8, no. 1, p. 3807, Feb. 2018, doi: 10.1038/s41598-018-22165-4.
[64] B. Majumder, V. S. Sankar, and H. Meena, “Design of an Artificially Engineered all Metallic Lens Antenna,” in 2020 IEEE
Asia-Pacific Microwave Conference (APMC), Dec. 2020, pp. 1072–1074. doi: 10.1109/APMC47863.2020.9331698.
[65] X. Ren, Q.-W. Lin, H. Wong, and W. He, “A Compact Gradient Refractive Index Metamaterial Lens for Endfire Fan-Beam
Radiation,” IEEE Antennas Wirel. Propag. Lett., vol. 20, no. 12, pp. 2339–2343, Dec. 2021, doi:
10.1109/LAWP.2021.3110646.
[66] P. Bantavis, “Study and design of compact quasi optical beam forming lens antennas in a parallel plate waveguide
technology for on boards satellite constellation missions,” These de doctorat, Rennes 1, 2021. Accessed: Jan. 06, 2022.
[Online]. Available: https://www.theses.fr/2021REN1S016
[67] F. Fan, M. Cai, J. Zhang, Z. Yan, and J. Wu, “Wideband Low-Profile Luneburg Lens Based on a Glide-Symmetric
Metasurface,” IEEE Access, vol. 8, pp. 85698–85705, 2020, doi: 10.1109/ACCESS.2020.2992653.
[68] A. Papathanasopoulos and Y. Rahmat-Samii, “A Novel Deployable Compact Lens Antenna Based on Gradient-Index
Metamaterials,” in 2019 IEEE International Symposium on Antennas and Propagation and USNC-URSI Radio Science
Meeting, Jul. 2019, pp. 625–626. doi: 10.1109/APUSNCURSINRSM.2019.8888779.
[69] A. Papathanasopoulos, Y. Rahmat-Samii, N. C. Garcia, and J. D. Chisum, “A Novel Collapsible Flat-Layered Metamaterial
Gradient-Refractive-Index Lens Antenna,” IEEE Trans. Antennas Propag., vol. 68, no. 3, pp. 1312–1321, Mar. 2020, doi:
10.1109/TAP.2019.2944546.
[70] C. Babayiğit, A. S. Evren, E. Bor, H. Kurt, and M. Turduev, “Analytical, numerical, and experimental investigation of a
Luneburg lens system for directional cloaking,” Phys. Rev. A, vol. 99, no. 4, p. 043831, Apr. 2019, doi:
10.1103/PhysRevA.99.043831.
[71] S. Shad, “STUDY AND DESIGN OF ARRAY AND BEAMSTEERING ANTENNAS FOR MILLIMETER WAVE BAND APPLICATIONS,”
- 150.
[72] W. Shao and Q. Chen, “Performance analysis of an all-dielectric planar Mikaelian lens antenna for 1-D beam-steering
application,” Opt. Express, vol. 29, no. 18, pp. 29202–29214, Aug. 2021, doi: 10.1364/OE.438182.
[73] Y. Amarasinghe, D. M. Mittleman, and R. Mendis, “A Luneburg Lens for the Terahertz Region,” J. Infrared Millim. Terahertz
Waves, vol. 40, no. 11–12, pp. 1129–1136, Dec. 2019, doi: 10.1007/s10762-019-00635-8.
[74] A. S. Andy and Y. Hao, “Flat Luneburg Lens at 0.24 THz for Antenna Beam Steering Applications,” in 2019 13th European
Conference on Antennas and Propagation (EuCAP), Mar. 2019, pp. 1–4.
[75] C. Bilitos, J. Ruiz-García, R. Sauleau, E. Martini, S. Maci, and D. González-Ovejero, “Design of Broadband Reflecting
Luneburg Lenses by Higher Symmetries,” in 2021 Fifteenth International Congress on Artificial Materials for Novel Wave
Phenomena (Metamaterials), Sep. 2021, pp. 053–055. doi: 10.1109/Metamaterials52332.2021.9577089.
[76] Z. N. Chen, Y. Su, and W. E. I. Liu, “Metantennas: Flat Luneburg Lens Antennas Using Transformation Optics Method
(TOM),” in 2020 International Workshop on Antenna Technology (iWAT), Feb. 2020, pp. 1–3. doi:
10.1109/iWAT48004.2020.1570609870.
[77] D. KVBL1 and K. J. Sankar, “An Optimized design for Luneburg Lens Scanning Antenna using PIN diodes for Beam-
Switching,” Int. J. Adv. Eng. Res. Dev..
[78] W. Shao and Q. Chen, “2-D Beam-Steerable Generalized Mikaelian Lens With Unique Flat-Shape Characteristic,” IEEE
Antennas Wirel. Propag. Lett., vol. 20, no. 10, pp. 2033–2037, Oct. 2021, doi: 10.1109/LAWP.2021.3102316.
[79] S. Ahdi Rezaeieh, A. Darvazehban, M. Khosravi-Farsani, and A. M. Abbosh, “Body-Matched Gradient Index Lens Antenna
for Electromagnetic Torso Scanner,” IEEE Trans. Antennas Propag., vol. 69, no. 10, pp. 6165–6174, Oct. 2021, doi:
10.1109/TAP.2021.3061593.
[80] J. Chen et al., “Highly efficient achromatic subdiffraction focusing lens in the near field with large numerical aperture,”
Photonics Res., vol. 9, no. 10, pp. 2088–2094, Oct. 2021, doi: 10.1364/PRJ.427322.
[81] S. Paul and M. J. Akhtar, “Novel Metasurface Lens-Based RF Sensor Structure for SAR Microwave Imaging of Layered
Media,” IEEE Sens. J., vol. 21, no. 16, pp. 17827–17837, Aug. 2021, doi: 10.1109/JSEN.2021.3084614.
[82] S. D. Campbell, D. H. Werner, Werner, and P. L, “Transformation Optics and Related Techniques for GRIN Lens Design,” in
2019 13th European Conference on Antennas and Propagation (EuCAP), Mar. 2019, pp. 1–3.
[83] A. Papathanasopoulos and Y. Rahmat-Samii, “Multi-Layered Flat Metamaterial Lenses: Design, Prototyping and
Measurements,” in 2020 Antenna Measurement Techniques Association Symposium (AMTA), Nov. 2020, pp. 1–6.
[84] M. Sedaghat, V. Nayyeri, M. Soleimani, and O. M. Ramahi, “Practical approaches to designing and fabricating flat lenses,”
- Appl. Phys., vol. 126, no. 1, p. 014901, Jul. 2019, doi: 10.1063/1.5095410.
[85] A. Paraskevopoulos, F. Maggiorelli, M. Albani, and S. Maci, “Radial GRIN Lenses based on the Solution of a Regularized Ray
Congruence Equation,” IEEE Trans. Antennas Propag., pp. 1–1, 2021, doi: 10.1109/TAP.2021.3111315.
[86] O. Manoochehri, A. Darvazehban, M. A. Salari, A. Emadeddin, and D. Erricolo, “A Parallel Plate Ultrawideband Multibeam
Microwave Lens Antenna,” IEEE Trans. Antennas Propag., vol. 66, no. 9, pp. 4878–4883, Sep. 2018, doi:
10.1109/TAP.2018.2845548.
[87] F. Maggiorelli, A. Paraskevopoulos, R. Giusto, M. Albani, and S. Maci, “Ray-tracing in Dielectric Inhomogeneous
Metalenses,” in 2021 15th European Conference on Antennas and Propagation (EuCAP), Mar. 2021, pp. 1–5. doi:
10.23919/EuCAP51087.2021.9411505.
[88] K. Liu, C. Zhao, S.-W. Qu, Y. Chen, J. Hu, and S. Yang, “A 3-D-Printed Multibeam Spherical Lens Antenna With Ultrawide-
Angle Coverage,” IEEE Antennas Wirel. Propag. Lett., vol. 20, no. 3, pp. 411–415, Mar. 2021, doi:
10.1109/LAWP.2021.3054042.
[89] M. Albani, I. Gashi, A. Paraskevopoulos, and S. Maci, “Analysis and Design of Inhomogeneous Dielectric Lens Antennas by
using Geometrical Optics,” in 2021 International Applied Computational Electromagnetics Society Symposium (ACES), Aug.
2021, pp. 1–4. doi: 10.1109/ACES53325.2021.00128.
[90] A. Paraskevopoulos, I. Gashi, M. Albani, and S. Maci, “Aperture efficiency limits in radial graded index lens antennas,” in
2021 Fifteenth International Congress on Artificial Materials for Novel Wave Phenomena (Metamaterials), Sep. 2021, pp.
319–321. doi: 10.1109/Metamaterials52332.2021.9577159.
[91] Q. Ma, C. B. Shi, T. Y. Chen, M. Q. Qi, Y. B. Li, and T. J. Cui, “Broadband metamaterial lens antennas with special properties
by controlling both refractive-index distribution and feed directivity,” J. Opt., vol. 20, no. 4, p. 045101, Feb. 2018, doi:
10.1088/2040-8986/aaacbf.
[92] D. Wang et al., “Cold-Sintered Temperature Stable Na0.5Bi0.5MoO4–Li2MoO4 Microwave Composite Ceramics,” ACS
Sustain. Chem. Eng., vol. 6, no. 2, pp. 2438–2444, Feb. 2018, doi: 10.1021/acssuschemeng.7b03889.
[93] A. Mohamed et al., “Design of a Graded Index Metasurface Lens for Gain Enhancement of Rectangular Dielectric
Resonator Antenna,” in TENCON 2019 – 2019 IEEE Region 10 Conference (TENCON), Oct. 2019, pp. 1444–1447. doi:
10.1109/TENCON.2019.8929548.
[94] H. Eskandari, S. Saviz, and T. Tyc, “Directivity enhancement of a cylindrical wire antenna by a graded index dielectric shell
designed using strictly conformal transformation optics,” Sci. Rep., vol. 11, no. 1, p. 13035, Dec. 2021, doi:
10.1038/s41598-021-92200-4.
[95] I. V. Soares, F. M. Freitas, and U. C. Resende, “Efficiency Enhancement in Mid-Range RWPT Systems by GRIN Metasurface
Lenses,” in 2021 IEEE Wireless Power Transfer Conference (WPTC), Jun. 2021, pp. 1–4. doi:
10.1109/WPTC51349.2021.9458020.
[96] H. Eskandari, M. S. Majedi, A. R. Attari, and O. Quevedo-Teruel, “Elliptical generalized Maxwell fish-eye lens using
conformal mapping,” New J. Phys., vol. 21, no. 6, p. 063010, Jun. 2019, doi: 10.1088/1367-2630/ab2471.
[97] S. S. Kulkarni, A. K. Baghel, and S. K. Nayak, “Graded refractive index metamaterial for enhanced far-field wireless power
transfer efficiency in S-band,” AEU – Int. J. Electron. Commun., vol. 138, p. 153859, Aug. 2021, doi:
10.1016/j.aeue.2021.153859.
[98] Z. Li, A. Olah, and E. Baer, “Micro- and nano-layered processing of new polymeric systems,” Prog. Polym. Sci., vol. 102, p.
101210, Mar. 2020, doi: 10.1016/j.progpolymsci.2020.101210.
[99] Z. Sabrina, R. Nathalie, and O. Rachid, “New Fabry-Perot cavity antenna with Inhomogeneous Dielectric PRS,” in 2018
International Seminar on Intelligent Technology and Its Applications (ISITIA), Aug. 2018, pp. 1–4. doi:
10.1109/ISITIA.2018.8710812.
[100] Q. Zhu, J. Huang, R. Ni, and M. Ma, “Switched Beam Lens Antennas Fed with Magneto-Electric Dipoles,” in 2020 14th
European Conference on Antennas and Propagation (EuCAP), Mar. 2020, pp. 1–4. doi:
10.23919/EuCAP48036.2020.9135654.
[101] W. wang, N. Garcia, and J. Chisum, “The Systematic Design of Non-commensurate Impedance Matching Tapers for Ultra
Wideband Gradient-Index (GRIN) Lens Antennas,” ArXiv210605425 Phys., Jun. 2021, Accessed: Jan. 05, 2022. [Online].
Available: http://arxiv.org/abs/2106.05425
[102] E. B. Whiting et al., “Topology Optimization of RF GRIN Lenses,” in 2020 IEEE International Symposium on Antennas and
Propagation and North American Radio Science Meeting, Jul. 2020, pp. 101–102. doi:
10.1109/IEEECONF35879.2020.9329804.
[103] Z. Yang et al., “Ultrawideband Antipodal Tapered Slot Antenna With Gradient Refractive Index Metamaterial Lens,” IEEE
Antennas Wirel. Propag. Lett., vol. 18, no. 12, pp. 2741–2745, Dec. 2019, doi: 10.1109/LAWP.2019.2950676.
[104] “A Solid Dielectric Lens Impulse Radiating Antenna for Missile Defense | SBIR.gov.” https://www.sbir.gov/content/solid-
dielectric-lens-impulse-radiating-antenna-missile-defense-0 (accessed Jan. 10, 2022).
[105] “An Improved Solid Dielectric Lens Impulse Radiating Antenna | SBIR.gov.” https://www.sbir.gov/content/improved-
solid-dielectric-lens-impulse-radiating-antenna-0 (accessed Jan. 10, 2022).
[106] C. A. Hamner and R. A. Spencer, “Conformal array, luneburg lens antenna system,” US8854257B2, Oct. 07, 2014
Accessed: Jan. 07, 2022. [Online]. Available: https://patents.google.com/patent/US8854257B2/en
[107] “FLAT Lens Antenna Technology for Compact UHF/VHF Antennas | SBIR.gov.” https://www.sbir.gov/node/1596359
(accessed Jan. 10, 2022).
[108] “Flat Lens Antenna Technology for Compact UHF/VHF Antennas | SBIR.gov.” https://www.sbir.gov/node/1860687
(accessed Jan. 10, 2022).
[109] “Parabolic Antenna Lens | SBIR.gov.” https://www.sbir.gov/node/2116535 (accessed Jan. 10, 2022).